Super-resolution fluorescence microscopy is microscopy that goes beyond the diffraction limit of light. It encompasses a series of techniques that have emerged over the last 30 years and exploded in the last decade, leading to a swift increase in the number of super-resolution imaging devices and publications across the research world, and biology in particular. However, there are barriers to their use in many situations.
This article illustrates those barriers and how they can be overcome by comparing super-resolution to the very widely used confocal microscopes. The goal here is to illustrate the differences in the philosophy behind their designs and show how novel techniques implement the best aspects of both.
Super resolution microscopy versus super-confocal systems: strength versus flexibility
Confocal microscopes: practical and versatile
Confocal microscopy is crucial in biological imaging because of its efficacy, ease of use, and versatility. With it, it is possible to obtain images of biological tissues with a lateral resolution of about 200 nm (depending on the wavelength and objective) and a depth resolution of about 500 nm with a penetration depth of up to roughly 0.5 mm.
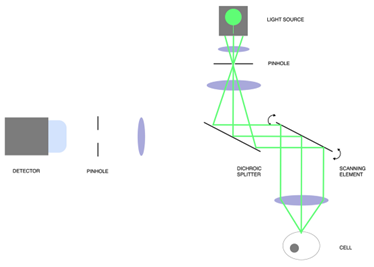
Figure 1 : Principle of confocal microscopy. In step 1, light is projected onto a single point of the sample through a pinhole. The emitted fluorescent light is collected through another pinhole and measured on a detector unit. The scanning element (mirrors or scanning disk) then rotate to measure the next pixel of the sample.
Confocal microscopes are easy to install and to use, generally requiring only a few hours to install or upgrade from wide-field microscopes and are relatively inexpensive. A new user can be trained to operate a confocal system in a single training session. At the same time, confocal microscopes can image a wide variety of samples and fluorophores – fluorescent proteins, antibodies, organic chemicals and more. Their short acquisition times mean it is possible to observe living tissue and cells at multiple frames per minute, or even per second.
Figure 2: A typical fluorescence microscope (Here a Nikon Ti Eclipse) with a confocal unit attached to it. The confocal unit is mounted on a camera port of the microscope, like any other camera would be.
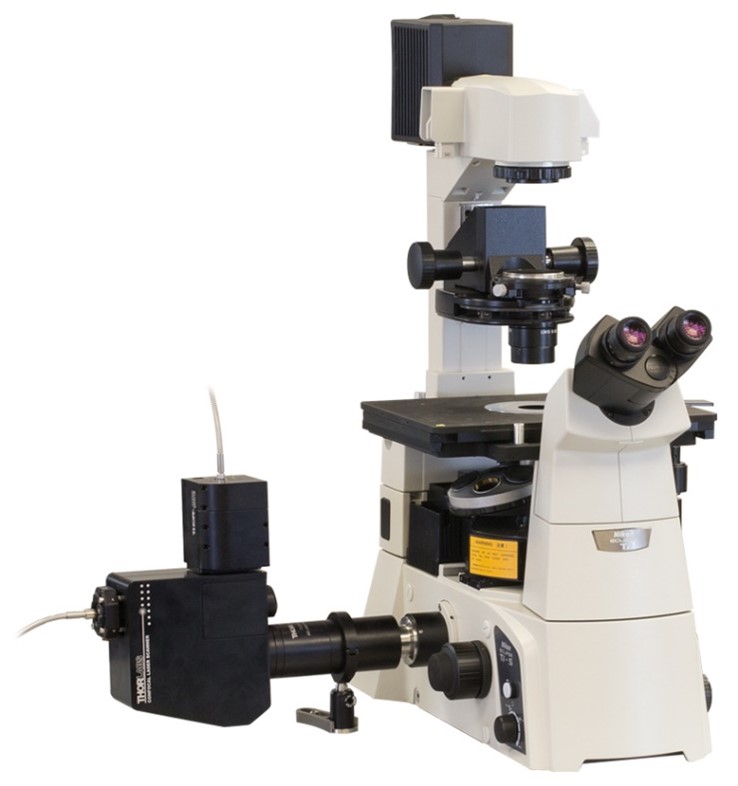
Power and complexity of super-resolution systems
The spatial resolution of a confocal microscope is limited by the diffraction of light. Super-resolution microscopy techniques have been designed to circumvent this limit by various optical means. For instance, Structured Illumination Microscopy (SIM) utilizes a patterned widefield illumination scheme. The patterns contain very thin lines of illumination alternating with darkness where the intensity differential is sharper than the diffraction limit of light. With robust algorithmic processing of the data, it becomes possible to resolve finer details at a resolution of 120 nm in most cases, but up to 60 nm in ideal cases with recently developed tools.
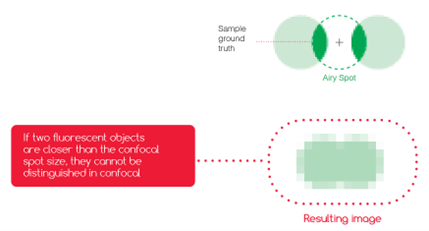
Figure 3: With scanning microscopes, such as confocal ones, the resolution (ie. the capacity to distinguish or “resolve” 2 close light sources) depends on the size of the spot you are scanning with. On this image, if the light spot (called the Airy spot in the case of confocal microscopy) is larger than the gap between the two fluorophores, it is not possible to separate the two spots, and they appear blurred together.
However, this technique requires relatively complex illumination systems. With thick samples, the diffusion of light caused by the sample can blur the finely tuned pattern, leading to both decreased resolution and additional artifacts in the acquisition.
Going deeper into super resolution imaging, PALM/STORM techniques boast the highest resolution of established super-resolution technologies at roughly 20nm. These technologies aim to separate fluorophores at the single molecule level by inducing stochastic blinking or on-off switching of the fluorophores and taking many thousands to tens of thousands of camera frames. Such an image can take between 5 and 15 minutes to acquire and involves multiple acquisition cycles, greatly increasing the photo-damage done to the sample and precluding any live-cell imaging.
In practice, super-resolution microscopy is often confined to imaging platforms that can afford to install and use those expensive machines, as well as train their users and offer continued on-site support staff.
Super-resolution devices do not replace confocal microscopes, but rather exist alongside them, providing biologists with additional insight into specific questions or high-quality images for publications. Since many biological questions do not even require super-resolution, the confocal microscope remains the first step in most imaging protocols.
Simplifying super-resolution: learning from confocal modalities
It often happens that a user will not want a better spatial resolution at the cost of increased complexity. Rather than a super-resolved imaging modality, biologists want a confocal super-resolved modality, with the same flexibility and ease of use as a confocal system, an improved resolution and maybe with additional benefits (such as improved signal-to-noise ratio). Those techniques that use a confocal geometry to attain super-resolution are quickly developing.
The oldest example of super-resolution confocal-based microscopies are STimulated Emission Depletion (STED) techniques. Those systems utilize a secondary laser beam that is shaped like a donut around excitation to de-excite fluorophores close to the excitation beam.
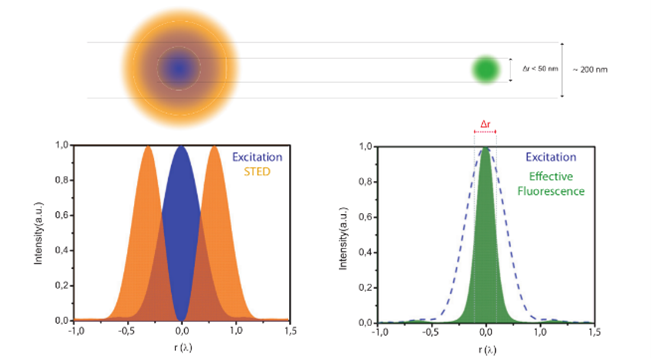
Figure 4: Principle of STED microscopy: Using a suppression laser as well as an excitation one, STED can create an effective scanning spot that is smaller than what a regular Airy spot would be.
In practice, this amounts to scanning with a smaller excitation point-spread function. This makes it possible to reach lateral resolutions of 50-70 nm with this technology but requires longer scanning times (the scanning time required for an image will increase quadratically with decreasing distance between scanning points) and requires specific fluorophores to function. Only limited wavelengths are available. All of this makes this technique more complex to use than an ordinary confocal microscope.
Another quite popular method is the AiryScan (Zeiss). This is a confocal device using a specific detector that is separated into different quadrants, making it possible to obtain redundant information on a scanning pattern. Thus, it is possible to obtain a lateral resolution of roughly 140nm (120 nm with deconvolution) at a wavelength of 488nm. Other techniques make use of deconvolution or repeated acquisitions to reach similar resolutions, while keeping the advantages of confocal microscopy.
Conical diffraction microscopy: confocal-configuration SIM
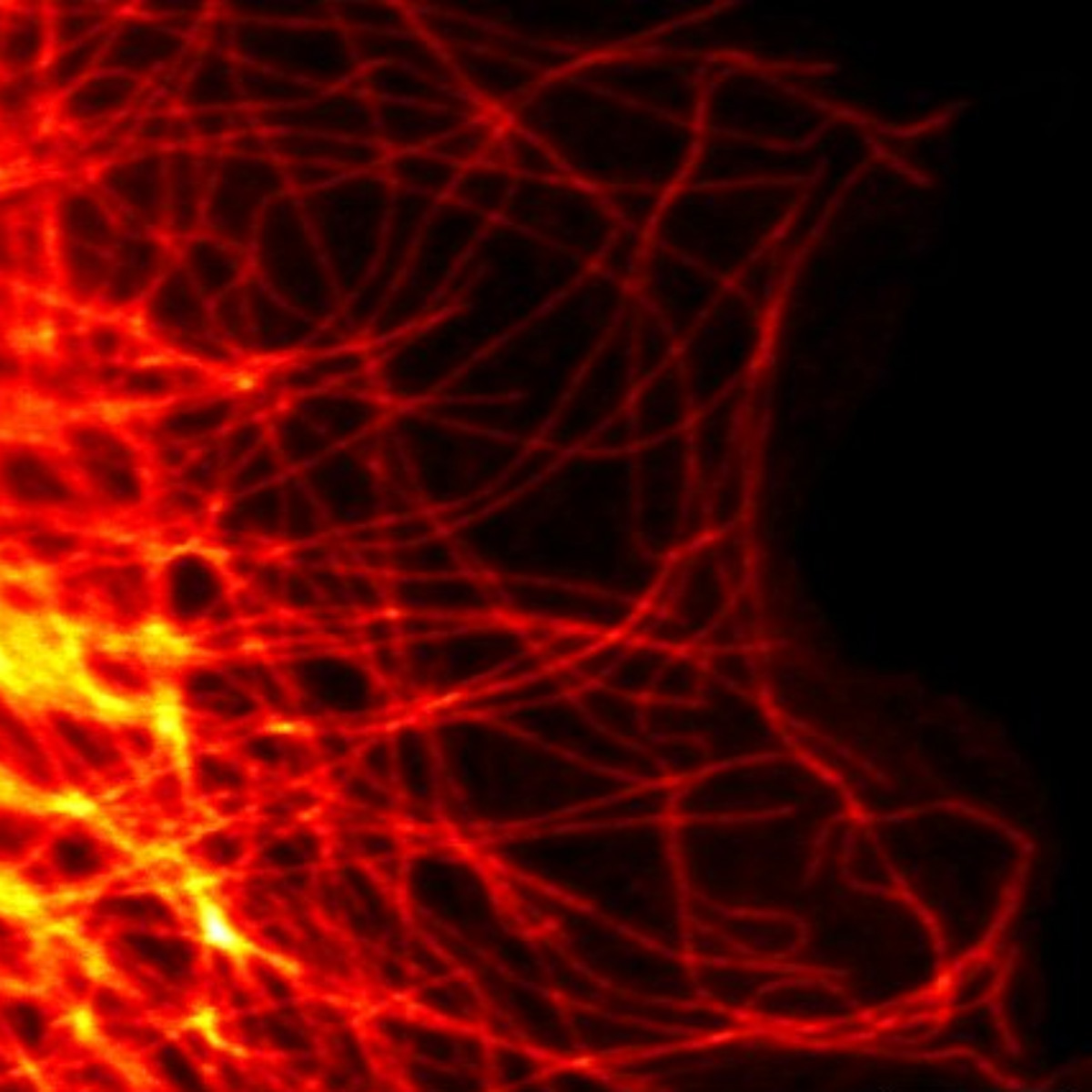
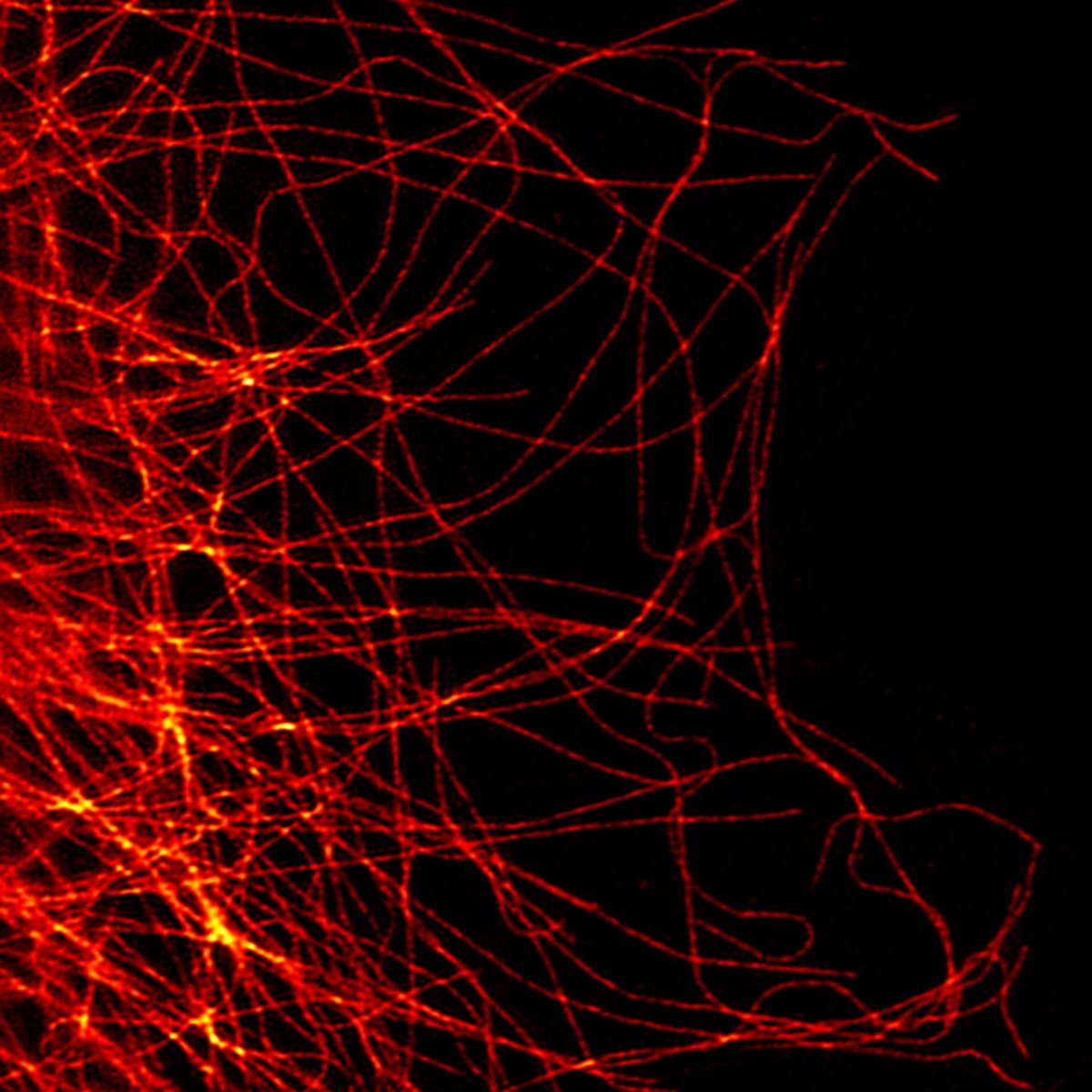
Structured illumination goes very well with confocal conceptions. This is what Telight does in our French branch, Bioaxial, in the form of the LiveCodim microscopy solution. A beam-shaping system modifies a laser beam to create a shaped light pattern in the form of two “half-moons” that offer a single extinction that is sharper than a classical Airy pattern. The sample is then scanned with this pattern, like a conventional confocal microscope. In a process analogous to a SIM device, but localized via raster scanning, it makes it possible to extract additional spatial frequencies from the sample, reaching a resolution of up to 90nm.
LiveCodim combines the advantages of confocal and structured illumination microscopy: it can run three-dimensional acquisitions (“Z-stack”), time-resolved measurements or image moving samples with no motion artifacts. LiveCodim microscopy can image any type of sample or fluorophore, requiring no change in sample preparation. The reduction of the photon budget minimizes the damage caused to samples, which is particularly useful for the imaging of living cells to preserve their fluorescence for repeated acquisitions.
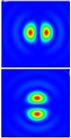
Figure 5: Typical half-moon patterns used in the LiveCodim imaging device
Super-resolution imaging systems focused on image resolution
As more and more super-resolution systems integrate the advantages of confocal geometries, we find ourselves with two different families of super-resolution imaging devices. Classical super-resolution systems offer the highest resolution possible, with cutting edge technologies now approaching the nanometer separation. They come however with severe limitations in terms of user complexity, sample thickness and preparation, imaging duration and device cost: they are “resolution-first.” Those systems – PALM/STORM, STED or MINFLUX, serve well as a core facility device, if a dedicated engineer is available to assist with imaging, sample preparation and image processing. In addition, not every biological question needs a 15 nm resolution to be resolved, and thus the need for those expensive machines is not ubiquitous: again, this is much better suited to large platforms that can mutualize costs.
Super-resolution imaging systems focused on biological imaging
The other family of super-resolution techniques – Airyscan, Re-scanning, certain STED and now LiveCodim – focus on minimizing constraints by having the same use-cases as confocal microscopy, but with better resolution and signal-to-noise ratios. Therefore, while they are unable to reach the same discriminating power, they can be applied to a much wider range of samples and situations. They do not need a dedicated platform or engineer to operate efficiently and can be used on any sample or situation. They create images quickly and gently. They have a “flexibility-first” mindset. Those “Super-confocal » devices do what a confocal does, super-resolved. As the confocal was before, they are the gateway to biological imaging, the first step in imaging that can be followed, if needed, by more resolved techniques.